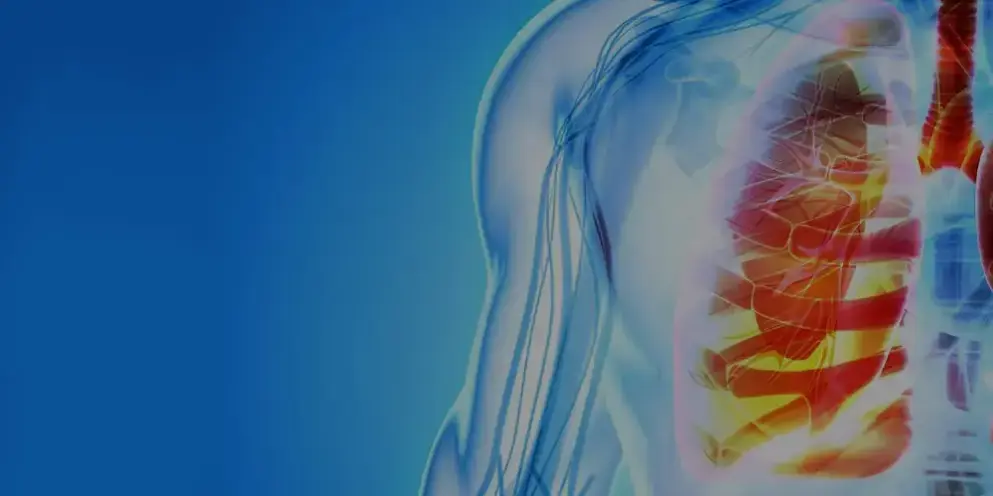
Respiratory
of interest
are looking at
saved
next event
Respiratory is a branch of medicine that specialises in the treatment of diseases that affect the respiratory system, it is also referred to as pulmonology.
Accredited eLearning module in chronic cough
Professor Kian Fan Chung discusses the diagnosis and management of chronic cough in adults in this EACCME® accredited eLearning. Receive 1 CME credit for successful completion.
Chronic cough: unmet needs, management and expert insights
Deep dive into the evolving treatment landscape for chronic cough and gain 1 CME credit with this EBAP accredited webinar
Respiratory deals with all aspects of the breathing system as well as being involved with intensive care medicine providing life support and mechanical ventilation. This medical speciality deals with asthma, chronic obstructive pulmonary disease, cystic fibrosis, allergic rhinitis and involved with smoking cessation and sleep apnoea.
It can also cover solid organ transplantation where replacement of the lung is needed for end-stage pulmonary disease from multiple causes.
In this section
Allergic Rhinitis
Containing relevant Learning Zones, guidelines, trials and news.
Asthma
Containing relevant Learning Zones, guidelines, trials and news.
Chronic cough
Containing relevant Learning Zones, guidelines, trials and news.
Chronic Obstructive Pulmonary Disease (COPD)
Containing relevant Learning Zones, guidelines, trials and news.
Cystic Fibrosis
Containing relevant Learning Zones, guidelines, trials and news.
Idiopathic pulmonary fibrosis (IPF)
Containing relevant Learning Zones, guidelines, trials and news.
Nasal polyposis
Containing relevant Learning Zones, guidelines, trials and news.
Respiratory Syncytial Virus (RSV)
Containing relevant Learning Zones, guidelines, trials and news.
Sleep Apnea
Containing relevant Learning Zones, guidelines, trials and news.
Smoking Cessation
Containing relevant Learning Zones, guidelines, trials and news.
Solid Organ Transplantation
Containing relevant Learning Zones, guidelines, trials and news.